“The enemy of my enemy is my friend,” reads an ancient proverb, and in the fight against antibiotic-resistant bacteria affecting human lives, it is proving true. Earlier this month, researchers from Baylor College of Medicine in Houston published a paper in the journal Scientific Reports, in which they described the use of bacteriophages to combat antibiotic-resistant bacteria. Read on to find out more about what phages are, and why we need them.
The Rise of the Superbugs
Resistance to antibiotics has existed since the development and widespread use of the first antibiotic, penicillin, in the 1940s. In fact Sir Alexander Fleming, the discoverer of penicillin, warned in his Nobel Lecture against the development of resistance that could result from easy access and subsequent incorrect usage of the antibiotic.
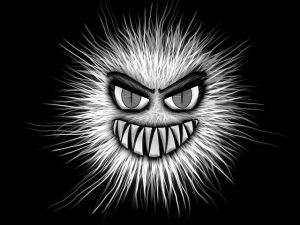
As resistance to penicillin inevitably spread, new generations of antibiotics were developed. Bacterial resistance eventually became problematic again, and in the decades that followed, many new antibiotics emerged. However, bacteria are becoming resistant to those as well. We call them “superbugs”, because not even the best weapons in our arsenal of drugs can kill them. Together with a lack of new antibiotic development, this has plunged us into what people have begun calling the “post-antibiotic era,” in which common injuries and infections can once again be life-threatening.
Examples of these superbugs include methicillin-resistant Staphylococcus aureus (MRSA), multi-drug-resistant Mycobacterium tuberculosis (MDR-TB), and carbapanem-resistant Enterobacteriaceae (CRE), like E. coli and Salmonella. CREs, in particular, are resistant to all or nearly all antibiotics available, and around 50% of hospital patients who suffer a blood infection caused by CREs die.
A dramatic example of a superbug infection was reported earlier this year. An elderly woman died of an infection caused by a CRE. She had been in a long-term trip to India, where she broke her femur some years back. She later developed a bone infection, and was hospitalized in India several times. After returning to the US, the woman sought medical care. She was hospitalized in Reno, where it was discovered that she had a CRE infection, in this case by Klebsiella pneumoniae. The bacteria was resistant to 26 different antibiotics, all of the ones that are available in the US. The woman went into septic shock and died shortly after. Even though infections like this one are not common, it is certainly an alarming reminder of what is already starting to happen.
Deaths attributable to antimicrobial resistance (from The Review on Antimicrobial Resistance) / CC BY 4.0
According to the Centers for Disease Control and Prevention, every year at least 2 million people in the United States are infected with antibiotic-resistant bacteria, and at least 23,000 people die from those infections every year. In The Review on Antimicrobial Resistance published in 2014, it is estimated that by 2050 the number of human deaths due to antibiotic-resistant bacteria will be 10 million.
How does resistance develop?
Some bacteria can be naturally resistant to antibiotics. Others may become resistant as a result of random mutations to their DNA. These bacteria then pass their resistance to their offspring. But, even more critically, they can pass their resistance genes horizontally to other bacteria through the exchange of plasmids, or mobile DNA elements. The horizontal spread of resistance can also happen from one species of bacteria to another.
When a bacterial population is exposed to an antibiotic, the susceptible bacteria die. However, if some of them have developed or acquired resistance, they will survive. The continued presence of the antibiotic acts as a selection pressure, killing all susceptible bacteria and allowing the resistant ones to thrive. Overusing antibiotics, as well as using them at a sublethal dose, promotes the development of resistance.
Factors contributing to the crisis
Overprescription of antibiotics, and the widespread lack of regulations regarding their use lead to excessive or unjustified human consumption. As we have seen, such abuse of antibiotics is an important cause of resistance development, but perhaps an even bigger threat is their agricultural use.
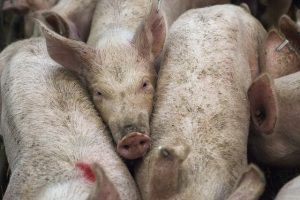
Although the practice has become increasingly controversial, antibiotics are still commonly used on farm animals, like pigs and chickens, to keep them healthy in crowded environments. They are also used to help the animals grow faster while consuming less food. This allows farmers to increase their yield substantially, but the great amount of antibiotics used, in total greater than that for human consumption, also means these farms are a prime source of resistant bacteria.
Antibiotic-resistant bacteria are present in the feces of these animals, and can become airborne and contaminate the environment. The presence of antibiotic-resistant bacteria has been confirmed in the gut of farm workers, and is also present in slaughterhouses, where the meat of these animals is processed for human consumption. This means that the bacteria can also reach consumers through contaminated meat. Among the resistant bacteria that have been linked to farm animals is Staphylococcus aureus (MRSA).
Adding to this, pharmaceutical companies are less inclined to develop new antibiotics than before. Developing these drugs has become a very expensive and lengthy process for companies, who must comply with very meticulous regulatory standards before being able to market a new drug. The speed with which resistance is developed in bacteria means that some antibiotics are soon obsolete, and many companies have turned their resources to the production of more profitable drugs.
Measures to restrict the use of antibiotics, both for human and animal use, have been established in some places. But as resistance continues to develop faster than new drugs can be created, scientists are beginning to look beyond antibiotics for potential solutions to this looming health crisis.
Bacterio…what?
Bacteriophages are viruses that infect bacterial cells and can destroy whole bacteria populations. They are widespread in nature wherever bacteria are present in high concentrations, like in the intestines of animals and sewages.
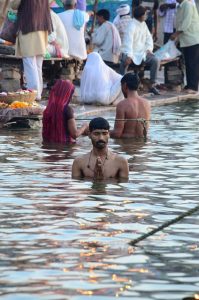
The first reports of bacteriophage activity date from the end of the 19th century. In 1896, Ernest Hankin wrote about the healing properties of the Ganges River in India, particularly against cholera. He attributed this property to an unidentified agent, which was small enough to pass through filters and could be inactivated by heat. Later on, in 1915, Frederick Twort described similar findings and suggested several agents that could be responsible, including a virus. However, Twort did not further pursue this line of research, and it was not until a few years later that Félix d’Hérelle, a French-Canadian microbiologist, began to study them seriously. He established that these agents were in fact viruses that could infect and kill bacteria. He named them “bacteriophages,” which means “bacteria eaters.” D’H’erelle also introduced the term page-therapy, or the treatment of bacterial infections with the use of bacteriophages.
Soon, phages started being used on patients, and were even commercially produced in Paris by what is now L’Oreal, and in the US by Eli Lilly. However, the studies analyzing the efficacy of phage therapy were controversial, and this, together with the discovery of antibiotics, led to a decline in interest in Western countries. Phages continued to be used in Eastern countries, particularly in Georgia and Poland, but most of the research was published in non-English languages and was largely unavailable to Western scientists.
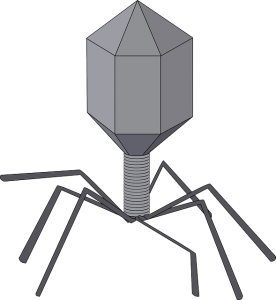
With the rise superbugs now considered one of the greatest threats to public health, and the lack of new antibiotics, interest in bacteriophages is again rising in the West. Bacteriophages have several advantages over antibiotics. Since superbugs and phages are both present in the environment, phages can evolve and adapt to their hosts in real-time, something antibiotics can’t do. They are also very specific for the type of bacteria they infect, which means that they won’t attack other beneficial bacteria, like our gut bacteria, the way antibiotics do. Furthermore, phages replicate inside their bacterial hosts, which means their dosage will increase inside the body as necessary.
The Research Findings
In the study published by the team from Baylor College of Medicine, the researchers sought to identify a phage for the extraintestinal pathogenic E. coli ST131 (ExPEC ST131). These bacteria are known to colonize the intestinal tract of people without producing symptoms, but can branch out and infect other organs, eventually leading to sepsis and even death. Strains of the type ST131 are resistant to multiple drugs, and are also very virulent. These bacteria are one of the main causes behind antibiotic-resistant E. coli infections in the United States.
The phages were obtained from goose, duck, and canine feces collected from local parks, and chicken feces from a farm. The researchers isolated the phages and tested them against several ExPEC ST131 strains taken from patients. Although no single phage was active against all bacterial strains, the combination of all the phages was.
The researchers then selected one of the promising phages and carried out experiments in mice to evaluate its performance in vivo. After injecting the mice with a virulent strain of ExPEC ST131, followed by a dose of the bacteriophage, they observed that phage-treated mice appeared healthier and had significantly lower bacterial counts than in those that didn’t receive phages.
Human patients with a compromised immune system, such as can be developed as a side effect of chemotherapy, are among the most susceptible to infection by superbugs. Antibiotics are routinely used on these patients, which in addition to promoting bacterial resistance, also eliminate useful gut bacteria , and are not useful with drug-resistant strains.
In this research paper, the this scenario was recreated by allowing a strain of ExPEC ST131 to colonize the intestines of two groups of mice. The researchers then gave the mice alternating daily doses of chemotherapy or phage for six days. Control mice received a placebo instead of the phages. After six days, they counted the bacterial load in several organs and found that it was significantly lower in all organs of phage-treated mice compared to those without phage therapy. The phages were able to lower the bacterial load to levels below what is considered fatal.
Conclusions
As the authors note, the most noteworthy aspect of this study is that they were able to demonstrate that phages against clinically-relevant strains of superbugs can be easily obtained from the environment, and applied in a short time. The fact that at least one of the phages obtained in this way was effective in a mouse model of bacterial infection with a compromised immune system was also encouraging.
Although no single phage was able to kill all the bacterial strains used, the combination of phages from all the sources resulted in killing all the strains. Therefore a cocktail of phages could be relevant in the clinic. The authors also propose the use of phages in combination with antibiotics. Although bacteria can develop resistance to phages, this might cause them to lose their resistance to antibiotics.
Phage therapy, although promising, has some challenges. As mentioned above, it is possible for bacteria to develop resistance to the phages, although phages can also change to adapt to their hosts. However, it has also been observed that the human immune system sometimes develops antibodies to fight the phages, making the therapy ineffective. Notwithstanding, phage therapy certainly seems like an interesting and so-far viable new weapon in our line of defense against bacterial pathogens.
Useful resources to learn more:
Loc-Carrillo, C. and Abendon, S.T. 2011. Pros and cons of phage therapy. Bacteriophage, 1(2), 111-114.
Review on Antimicrobial Resistance. Antimicrobial Resistance: Tackling a Crisis for the Health and Wealth of Nations. 2014.
Sulakvelidze, A., et al. 2001. Bacteriophage Therapy. Antimicrobial Agents and Chemotherapy, 45(3), 649-659.
Ventola, C.L. 2015. The Antibiotic Resistance Crisis. Pharmacy and therapeutics, 40(4), 277-283.