The king was down! Dropping everything, he had followed his master into the rain, through the throngs of soldiers, into the confusion of battle. The air stank of death. The king was bleeding profusely, there was no time to lose. Working quickly, they applied the venom over the wound and …the bleeding had stopped. The King would live. Sitting under the stars that night he wondered, as he had so many times before…how could something so deadly, give life? His own brother had died as a child from the snake’s bite and yet, King Mithridates lived because of it.
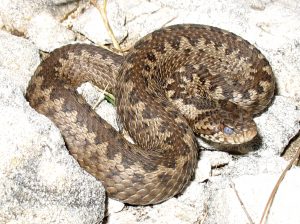
It was the year 67 B.C. and king Mithridates VI of Pontus was waging war against the Roman Republic. He became injured in battle and was bleeding heavily. His Agari healers, members of a Scythian tribe that traveled with his host as physicians, saved his life by applying the venom of the meadow viper (Vipera ursinii) to his wound to stop the bleeding (1).
Fast forward to the 21st Century, in a different part of the world…It was a game of hide and seek. Scouting the terrain with anxious eyes, she finally found the perfect hiding spot. They will never find me here, she thought, grinning . As she knelt behind the termite mound, careful not to make a sound, she felt a sting on her shoulder. Pain gave way to horror as she turned and discovered the snake. And not just any snake, a black mamba. It too had been hiding behind the termite mound, basking. She alerted the kids and they ran to their mother, but it was too late. The bite of the black mamba, known as the kiss of death, can kill a grown man in under an hour…the 13 year old girl never stood a chance (2).
Snakes. They terrify us and attract us at the same time. Since times immemorial, snakes have been a part of the human culture, both feared and revered. Their ability to strike down even the strongest among us inspires terror and respect. And yet, the healing power of their venoms has been recognized since ancient times. Indeed, how can something so deadly save lives? To answer that question, we must first clarify what snake venom is.
What is snake venom?
Snake venom is a modified saliva used in hunting and/or defense, which is injected into another organism and produces a toxic effect (3). It is made up of proteins and smaller peptides—which are responsible for most of the symptoms of envenomation—, carbohydrates, and other nonproteic compounds. Venom is designed to quickly immobilize or kill prey and predator. The components that make venom effective, called toxins, are very specialized, and have high biological activity (meaning they have a high capacity to affect our bodily functions). They can have an effect on many different body systems, including the central and peripheral nervous systems, and the cardiovascular system.
Snake venoms demonstrate a wide range of diversity. Composition can vary according to species, age, and geographical location. Differences have even been found between individuals in the same litter (4). Although not all sources of variation are understood, perhaps the most obvious one is diet, since venoms are specialized to target the snake’s preferred prey.
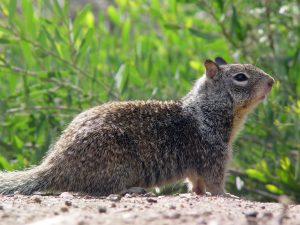
The genes that encode venom toxins can undergo rapid evolution, and thus permit snakes to adapt and consume a great variety of prey (3). In some cases the “arms race” between prey and predator can be clearly observed. For example, California ground squirrels are typically heavily hunted by Northern Pacific rattlesnakes. In response to this, squirrels have developed varying levels of resistance to rattlesnake venom. In areas where rattlesnakes are common, components in the blood of adult ground squirrels have been found to neutralize toxins in the snake venom. However, this resistance is lacking in squirrel populations living where rattlesnakes are not as common. To counter it’s prey’s resistance, rattlesnake venom and preying habits have had to adapt over the years (5).
Medicinal Potential
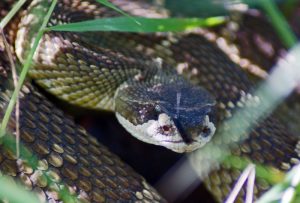
“Northern Pacific Rattlesnake” by Allie_Caulfield / CC BY 2.0*
Venom has evolved to attack and disable both predators and prey in a short amount of time. To do this, it contains toxins capable of identifying and attacking key targets that are responsible for the control of physiological processes. Often, these same targets are implicated in disease conditions. By studying the way toxins act, scientists can learn more about how our own systems work, and discover new targets in the development of cures for different diseases.
Because of the immense variability in venom composition, as well as the rapid evolution that venoms undergo, they are like a goldmine of potential drugs. Takacz and Nathan report that the diversity of venomous animals on Earth ranges from 100,000 to 170,000, representing more than 20 million toxins. Of those, only around 1000 have been carefully studied by scientists, and this has resulted in 15 different drugs. Animal venoms have a vast potential for the development of therapeutic drugs, which largely remains untapped.
Once a toxin has been identified for its potential medicinal effect, it can be either used as is, or it can serve as a template to create a mimetic compound. Mimetic compounds are molecules that are designed to behave in the same way as the original. They can have several advantages over the original molecules, including improved bioavailability, which can make it possible for them to be taken orally, since most venom toxins need to be injected. Moreover, the variation of venom composition between specimens makes producing a product of consistent quality through purification of toxins directly from crude venom, difficult. However, mimicking the complex structure of toxins is a challenging endeavor (3).
Venom toxins can be used in diagnostics, and as therapeutic agents. As of 2014, Takacs and Nathan reported 15 venom toxins used in diagnostics tests, all of them obtained from snake venoms. Out of the 15 therapeutic drugs derived from animal venoms, 8 of them come from snakes. Drugs derived from snake venoms are indicated for different medical conditions, including hypertension, cardiac failure, acute coronary syndrome, and acute cerebral infarction. They are also used as defibrinating agents and for the treatment of hemorrhage during surgery (3).
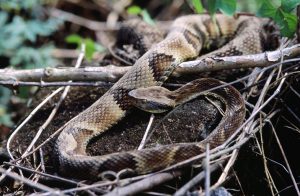
A toxin from the venom of the South American pitviper Bothrops jararaca was used to produce ACE inhibitors, a class of drugs used to lower blood pressure in patients with hypertension and heart disease.
Cobra venom factor (CVF) is a compound that has been used to reduce the chance of immunological rejection in transplant patients. It is found in the venom of different species of cobra, and acts by depleting the immune complement system (4).
Antibacterial properties have been observed in the venom of some snakes, including the inland taipan, considered to have the most toxic venom of all snakes. Also, two components of the venom of the king brown snake (Pseudechis australis) were found to be 70 and 17.5 times more effective than the antibiotic tetracycline in an in vitro assay (4).
Two interesting compounds that are currently being developed into therapeutic drugs by the company Theralpha are derived from hannalgesin from the king cobra (Ophiophagus hannah), and mambalgins. Both are part of the family of three-fingered peptides found in elapid venoms, and have an annalgesic effect greater to or similar to morphine, which is considered the gold standard in pain relief.
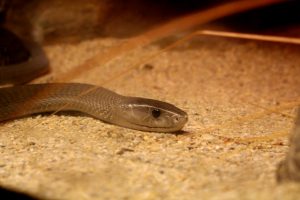
Mambalgins are peptides found in the venom of the Black Mamba (Dendroaspis polylepsis). What is especially interesting about these molecules is that they apparently act through an entirely different pathway than morphine, and lack the side effects of this drug, including creating tolerance (6).
Mambalgins work by inhibiting acid-sensing ion channels (ASICs) that are found on nociceptors. Nociceptors are neurons that sense pain stimuli. By interfering with the ASICs, mambalgins effectively block the sensation of pain. It is not clear why mambalgins are present in black mamba venom, but it has been suggested that their function is to prevent prey from fleeing (7).
An example of how similar toxins can have entirely different effects are two peptides present in the venom of the Texan coral snake, which also interact with ASICs. In this case, they overstimulate nociceptors by causing the ion channels to remain open. Overstimulation results in continuous pain signaling, which translates into the intense, burning pain which is a characteristic symptom of the bites of this snake (7;8).
How are venom toxins studied?
Observation of the effects of envenomation is one way in which potentially useful toxins can be identified. For example, when looking for compounds to help prevent blood clot formation, one could look for venoms that cause the blood to flow freely without clotting. However, a disadvantage of this approach is that compounds that are present in small concentrations might not contribute to the envenomation symptoms, and may be overlooked. A different approach is to screen a variety of venoms by dividing them into different fractions, and then testing out each fraction to observe its effects (4).
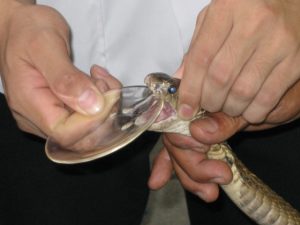
One of the main bottlenecks in the process of identifying useful snake toxins is the low volume of venom that can be obtained through milking. Milking is the process through which venom is extracted. It involves making the snake bite on a container and massaging its venom glands to help the venom flow. In a typical milking session, most medically relevant snakes produce around 100-200 mg of venom, with some very large snakes being able to produce up to more than a gram (4). This is a relatively straightforward, albeit very risky, procedure with front-fanged snakes like those of the cobra and viper families. However, with rear-fanged snakes, which have a less developed venom injection apparatus, venom is harder to extract.
The amount and ease with which venom can be milked is one of the reasons that, traditionally, venom screening has been relegated only to the venom of those snakes of medical importance. Rear-fanged members of the colubrid family were generally thought to have venoms of low toxicity (except for a few species, like the Boomslang), and because of this their venoms have not been so widely studied. However, scientists have realized that even though most of these snakes do not pose a threat to humans, they do produce potent toxins that can be of interest. However, the small amount of venom available still represents a challenge (4).
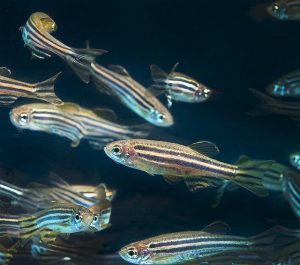
“Zebrafish” by Oregon State University/ CC BY-SA 2.0 The use of zebrafish has been proposed for the initial screening of venom toxins.
Typically, snake venom components are tested on mice. However, during the initial screening of numerous venom fractions, the large amounts of volume needed to treat mice may be prohibitive. For this reason, the use of other organisms, particularly zebrafish, has been proposed (4). The use of zebrafish has several advantages, including the much smaller volume of venom needed, as well as the possibility to observe how the toxins affect embryo development (thanks to their transparent eggs and embryos). The targets affected by snake toxins are largely conserved across many organisms (3), which means that although zebrafish will never be able to completely replace testing in mammals, they could be used for initial venom screening.
Another strategy that has improved the study of venom toxins is the creation of toxin libraries. Dr. Zoltan Takacs, a hungarian-born toxinologist that specializes in collecting and studying snake venoms, is the co-developer of the Designer Toxins technology. This is a tool designed to create libraries of toxins which are screened for action on a specific target. This makes it more efficient to identify potential therapeutic agents. Takacs is also the founder of the World Toxin Bank, which “is a biotechnology initiative exploring the toxin arsenal in Earth’s venomous animals for medical applications.”
Conclusion
Snake venoms represent a rich source of potential therapeutic agents. However, investigating their benefits involves hard and laborious work, including risky fieldwork. According to Dr. Takacs, it takes approximately 25 years since a toxin is first discovered to develop a drug for human use. Unfortunately, the populations of many species of snake have been dwindling over the last years (9). Snakes are considered undesirable by many, but perhaps we can gain a new perspective if we consider that loss of snake biodiversity also means loss of many possibly life-saving compounds.
If you want to learn more, you can check out the references:
1 Mayor, A. 2011. The Uses of Snake Venom in Antiquity. In Wonders and Marvels.
2 BBC, 2009. Documentary. Black Mamba, White Witch.
3 Takacs, Z., and Nathan, S. 2014. Animal Venoms in Medicine. Encyclopedia of Toxicology, 3rd ed. 252-59.
4 Vonk, F.J., Jackson, K., Doley R., Madaras, F., Mirtschin, P.J., and Vidal N. 2011. Snake venom: From fieldwork to the clinic. Bioessays, 33(4), 269-79.
5 Biardi, J.E., Chien, D.C., Coss, R.G. 2005. California ground squirrel (Spermophilus beecheyi) defenses against rattlesnake venom digestive and hemostatic toxins. Journal of Chemical Ecology, 31(11), 2501-18.
6 Diochot, S., Baron, A., Salinas, M., Douguet, D., Scarzello, S., Dabert-Gay, A.S., Debayle, D., Friend, V., Alloui, A., Lazdunski, M., Lingueglia, E. 2012. Black mamba venom peptides target acid-sensing ion channels to abolish pain. Nature, 490, 552-55.
7 Bladen, C. 2013. Taking a bite out of pain. Channels, 7(2), 69-70.
8 Bohlen, C.J., Chesler, A.T., Sharif-Naeini, R., Medzihradszky, S.Z., King, D., Sánchez, E.E., Burlingame, A.L., Basbaum, A.I., and Julius, D. 2011. A heteromeric Texas coral snake toxin targets acid-sensing ion channels to produce pain. Nature, 479, 410-14.
9 Reading, C.J., Luiselli, L.M., Akani, G.C., Bonnet, X., Amori, G., Ballouard, J.M., Filippi, E., Naulleau, G., Pearson, D., and Rugiero, L. 2010. Are snake populations in widespread decline? Biology Letters, 6(6), 777-80.
And the resources listed below:
King, G.F. Ed. 2015. Venoms to Drugs: Venoms as a Source for the Development of Human Therapeutics. The Royal Society of Chemistry.
How nature’s deadliest venoms are saving lives
*Image scaled and reduced to optimize for web viewing
I knew it, I was waiting for an article about snakes in your blog, what a nice piece of scientific literature!!, congrats!